February 16, 2005 – The development of the amniote brain is a territorial affair, with individual neurons vying for limited neurotrophic resources, groups of cells staking out turf and erecting various molecular signposts and fences to protect their claims, and entire neuronal populations that arise and fade away over the life of the animal. While this may sound like unneighborly behavior, the borders drawn in these developmental processes are of great importance in establishing the regions that give the brain its diversity of function.
In zebrafish, two distinct populations of neurons, primary and secondary, manifest during its ontogeny, with the primary neurons playing the vital role of enabling the larval fish to move, sense and respond to their environment immediately after hatching. These primary neurons form from regions known as proneuronal domains, which appear as three longitudinal stripes on the dorsal side of the embryo. The proneuronal domains can be visualized by staining for marker genes characteristic of proneural cells, such as neurogenin1 (neurog1), olig2 and pnx, and the mechanisms by which cells in these regions are specified to neuronal fates are fairly well understood. However, the process responsible for delineating the interproneuronal stripes, in which no proneural gene expression is observed, has remained largely a mystery.
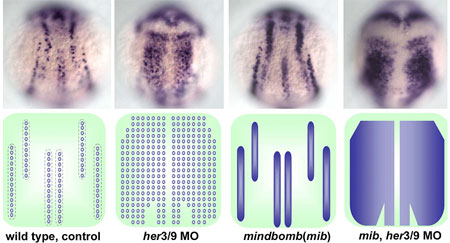 |
Changes in expression of the neuronal marker gene, elavl3, in her3/9 morphant, mind bomb and combined phenotypes. Wildtype shown on left. |
Young-Ki Bae and colleagues in the Laboratory for Vertebrate Axis Formation (Masahiko Hibi, Team Leader) set out to determine what might be responsible for this pattern of alternating proneural gene expression. Studies of proneuronal development in Drosophila have shown that cells adjacent to proneuronal neighbors begin to express a pair of genes, Her/Hes (forhairy and enhancer of split-related) genes, which encode transcriptional repressors to block neurogenesis in the cells in which they are expressed. Similar roles have been demonstrated for related families of genes in vertebrates, including mouse and amphibian (Xenopus). In some contexts, the activation of proneural suppressors occurs downstream in the Notch pathway, while other studies have shown that the position and width of proneuronal domains and intervening non-proneuronal bands seem to be regulated by positional information.
Bae et al looked at a pair of zebrafish Her/Hes genes, her3 and her9, which are expressed in the three inter-proneuronal domains running parallel down the dorsal aspect of the zebrafish posterior neurectoderm. Comparisons of her3 and her9 expression patterns against those of known proneuronal markers showed that both were restricted to the regions between proneuronal stripes, with her3 showing a more limited range. They tested the effects of altered Bmp signaling on the expression of these genes by tracking their expression in mutants in which the interproneuronal domains spread as a result of interference with the Bmp pathway. These mutants showed corresponding expansions of her3 and her9, indicating that the expression of these genes responds, either directly or indirectly, to positional information set up by Bmp.
They next examined the role of Notch signaling, which is known to play a role in the regulation of Her genes in many contexts in zebrafish development. Surprisingly, however, the team found that neither a mutation of the gene, mind bomb, which is involved in the activation of Notch signaling, nor the introduction of a chemical inhibitor of the Notch intracellular domain, nor an antimorphic form of the Delta protein had any effect on her3 or her9 in early neurogenesis.
Asking whether the interproneuronal regions might be determined secondarily by the proneuronal domains, the Hibi team tried misexpressing the proneuronal gene neurog1. They found that although neurog1 could induce ectopic proneuronal domains, it had no impact on her9. (It did reduce her3 expression, possibly through the upregulation of the gene for the Notch receptor, delta.) Similarly, morpholinos for neurog1 and olig2, which inhibit the development of various primary neurons, had no effect on either her3 or her9.
To investigate the function of her3 and her9, Bae looked at phenotypes in embryos in which these genes’ functions had been knocked down by antisense morpholino oligonucleotides, and discovered that loss of her9 function resulted in ectopic expression of proneural signature genes in interproneuronal domains, as did knockdown of her3, although its effect was more spatially constrained.Combined knockdown of her3 and her9 function led to the loss of interproneuronal domains and induced ubiquitous neurogenesis in the neural plate. Furthermore, the inhibition of both her3/her9 function and Notch signaling led to ubiquitous and homogenous expression of proneural and neuronal genes in the neural plate.
These findings led the lab to propose, in a report published in the journal Development, a new model for the patterning of the embryonic zebrafish brain in which Notch and her3/her9 play distinct roles in the organization of the alternating pro- and interproneuronal bands and neural specification. As previous reports have shown, Notch signaling works to limit the number of cells that adopt a neural fate by lateral inhibition within a given proneuronal domain, but it is the prepatterning of the neuroectoderm by her3 and -9 that give the zebrafish its earliest set of stripes.
|