Getting inside invagination |
 |
 |

November 16, 2007 –Most organ systems of animals are derived from a sheet-like layer of cells, the epithelium, that enclose the early embryo. Each organ is segregated from distinct primordial regions consisting of thickened epithelium, known as placodes. Orderly internalization of the placode segregates and shapes organ primordia into unique morphologies in the process called invagination. During invagination, the epithelial cells coordinate their movements such that they internalize through a clearly defined pore or furrow in a precise time course. The molecular machinery that enables cells at the placode to communicate with each other, however, is unclear. It has also remained a mystery how the cells in a flat sheet of epithelium could be squeezed through a narrow pore to the inside of the body cavity.
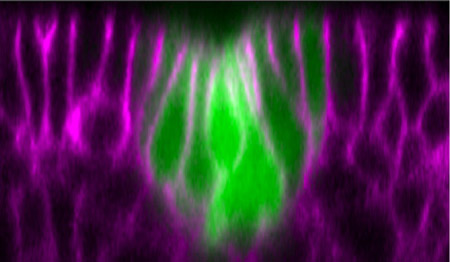 |
An optical cross section of the Drosophila tracheal placode (green) in an early stage
of invagination; cells showing apical constriction. Cell boundaries marked with magenta.
|
New image analyses by Mayuko Nishimura and colleagues in the Laboratory for Morphogenetic Signaling (Shigeo Hayashi; Group Director) performed on the Drosophila embryo have now shown that the invagination of the Drosophila tracheal placode involves rearrangements of cells in which the distribution of the non-muscle myosin motor protein is controlled by the EGFR pathway. .
The Hayashi group used time-lapse imaging of the shape changes, movement and proliferation of GFP-tagged primordial tracheal cells, allowing them to compare changes in the distribution of cytoskeletal F-actin and myosin. Approximately one hour prior to invagination, the cells stopped dividing and a circular arrangement of a few cells began to narrow at their apical surfaces and shift basally, leaving a depression in the center of the circle (the tracheal pit). Tracing the cells in the crucial minutes before invagination showed that the intercalation process by which migrating cells displace others proceeds in a polarized fashion, with cells moving toward the center of the prospective placode. This intercalation event explains, at least in part, why cells in the flat sheet of epithelium can be squeezed into a narrow tracheal pit.
Nishimura’s image analyses also revealed that groups of four to six cells immediately surrounding the placode often arrayed themselves in such a way cells which had once had zigzag contours began to form smoother, arc-like boundaries (a process the group has dubbed “boundary smoothing”). By tracing the accumulation of myosin, a protein linked to contractile activity at cell junctions, they found that although myosin expression started at generally low levels, it was upregulated in cells with shrinking junctions during intercalation and, later, particularly in those undergoing boundary smoothing.
Suspecting that the signaling pathway mediated by the tyrosine kinase receptor epidermal growth factor (EGFR), which has previously been implicated in tracheal invagination, might be involved in boundary smoothing and intercalation, Nishimura et al. used an antibody against the downstream factor ERK, a MAP kinase, to visualize EGFR signaling during the formation of the tracheal primordium. In the 30-minute period before invagination, they saw the ERK protein move from nuclear and cytoplasmic sites to the apical cortex near the surface of the cell, before fading at the onset of invagination. Interestingly, this pattern of ERK distribution under the control of EGFR appears as a ring expanding outwards from a center that spatially coincides with the tracheal pit where invagination later takes place.
Studies of flies bearing mutations for various genes associated with EGFR signaling showed that this pathway is required for both the apical constriction that creates the tracheal pit and for tracheal invagination to occur at the proper timing. In Egfr mutants, the onset of invagination was delayed and the apical constriction significantly impaired, and similar, though less pronounced, phenotypes were seen in mutants for an EGFR-activating ligand and a target factor that functions downstream of the EGF receptor. These mutants also exhibited defects in the cell arrangements responsible for controlling the location of the tracheal pit, where invagination occurs; in contrast to wildtype, mutants frequently developed ectopic or supernumerary tracheal pits.
Looking next to a possible regulatory relationship between EGFR signaling and myosin, the group examined cells labeled for both myosin and ERK. They found that the boundaries of cells with high ERK expression correlated strongly with the arcs of high-myosin cells, and that such arc-like rows were absent in Egfr mutants. Misexpression of an EGFR-activating ligand in normally flat epithelium produced an interesting mimic of the invagination phenotype of epithelial depression and myosin accumulation at the borders of neighboring cells in which one has high, and the other, low, ERK activity.
“In this study, we were able to show a functional link between EGFR signaling and myosin, a major motor protein causing cell contractility,” says Hayashi. “Although the myosin-dependent force in each of the cells is small, its coordination in arc-like cell rows is likely to generate a directed force that drives net movement of the placode. This wave-like propagation of EGFR activity explains how cell contractility in a large field of epithelia remains coordinated. And, as myosin activity is a general requirement for placode invagination and body axis elongation in both insects and vertebrates, we anticipate that a similar wave-like mechanism of signal propagation will be found in other organ systems.”
|