Cerebral cortical tissue from ES cells |
 |
 |
November 4, 2008 – The cortical regions of the brain are structurally complex and functionally important, providing the neural substrate for many of the brain’s highest functions, such as language, memory, emotion and thought. The cortex begins to form from the anterior end of the neural plate, and is characterized by its laminar structure in which neurons born at different developmental stages migrate to different cortical layers, each with its own functions and circuitry. Given this highly stratified and interconnected three-dimensional architecture, corticogenesis would at first blush seem to require involve myriad, complex processes difficult to reproduce in vitro.
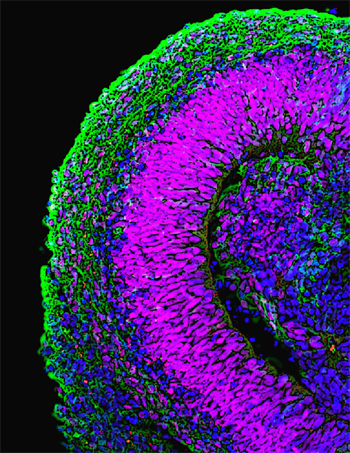 |
Immunostaining of cross-sectioned cortical tissue generated from human ES cells in vitro. |
|
Recent work by Mototsugu Eiraku and others in the Laboratory for Organogenesis and Neurogenesis (Yoshiki Sasai; Group Director) reveals a surprisingly straightforward method for generating three-dimensional aggregates of multiple, spatiotemporally organized cortical neuron types from embryonic stem (ES) cells. The report, published in Cell Stem Cell, opens new insights into the genetic control of cortical development, but also provides a technique for generating different types of cortical neurons for use in the study of pathogenesis and drug discovery.
The Sasai lab had previously developed an ES cell culture method, known as serum-free culture of embryoid body-like aggregates (SFEB), which they last year to induce the generation of forebrain neuronal precursors that expressed cortical marker genes from mouse embryonic stem cells. By tweaking the SFEB protocol, causing the cells to re-aggregate more quickly after dissociation, Eiraku found he could increase the efficiency of cortical differentiation. Naming the modified method, SFEBq (for quick-aggregation), he found that not only did the technique yield higher percentages of cortical precursors, but also that these reliably went on to form polarized neuroepithelial structures.
The group next cultured the mouse ES cell-derived cortical cell masses within forebrain slices, and found that the explants preferentially migrated into pallial regions, as would be expected from the normal destination of such cells in vivo. When they transplanted the same cells into the brains of newborn mice, they found that within four weeks they had developed into pyramidal neuron-like cells with typical morphology and patterns of axon extension.
To study the functionality of the cells, Eiraku looked at spontaneous neuronal activity (as measured by surges of calcium ions) in the cells, and found that this was sensitive to known chemical activators and inhibitors of such activity, indicating that it depended on local synaptic connections. Neonatal cortex is known to exhibit a unique form of coordinated activity, characterized by an oscillating wave of Ca2+that washes across relatively large fields of neurons. Surprisingly, even this calcium wave is recapitulated in the ES cell-derived cortical tissues, showing that they mimic the properties of the neonatal cortex to a remarkable extent.
During embryogenesis, the cortex undergoes regional specialization as the result of molecular signals emitted by surrounding tissues, such as FGF, which induces rostral cortical regions, and Wnt and BMP, which are required for the specification of the choroids plexus and cortical hem. When treated with these factors in culture, the SFEBq-differentiated cortical progenitor-like cells responded to these signals similarly to their embryonic counterparts, giving rise to the apposite neuronal types.
Eiraku and colleagues next sought to find out whether the mouse ES-cell derived cells would follow the stereotypical “inside early, outside late” birthing pattern observed for cortical neurons in vivo. Watching the sequence of marker expression in their cultured cortical-like cells, they found that they closely mirrored the temporal changes in gene expression seen in the embryonic cortex.
Having examined the temporal aspects of birthday-dependent differentiation, the Sasai group turned to the structure of the cells, which form hollow rosette-like clusters in suspension culture. Here again, they found that these rosettes comprised at least four distinct zones (as shown be gene expression), mimicking certain structural aspects of the early cortex.
Having extensively studied the ability of mouse ES cells to give rise to cells closely resembling cortical neurons, Eiraku et al. tested whether the SFEBq approach would work for human ES cells as well. Although the cells did not cluster into rosettes, forming larger, mushroom-shaped aggregates instead, the resulting continuous neuroepithelia expressed genetic markers characteristic of cortex and showed some of the regionalization seen in the mouse ES-derived structures.
“The most surprising finding for us was that a patternless clump of cells can reorganize itself into something that so closely resembles cortical tissue, so we’re eager to find out more about the role of such self-organization in brain development,” says Sasai. “What’s more, the fact that we were able to derive not just individual cell types, but functional tissue from ES cells may have a real impact in terms of both its medical significance and its value in drug discovery.”
|