March 1, 2005 – The ear’s architecture is ornate, with the three distinct components of outer, middle and inner ear coordinating to enable the senses of hearing, motion and balance. These otic subunits arise via separate genetic programs, the foundations of which are laid down very early in the embryo’s development. The inner ear, which grows to become home to populations of signal-transducing hair cells used in audition and maintaining equilibrium, is a marvelously intricate and multifunctional structure. It derives from a small patch of embryonic tissue known as the otic placode, a thickened disc that appears in an ectodermal region that would otherwise be destined to become skin.
Studies in chicken and mouse have shown that the specification of this placode is the outcome of a pattern of tissue interactions between tissues from at least two of the three embryonic germ layers, mesoderm and ectoderm. This previous work demonstrated that, in both species, the adjacent regions of head mesoderm and neural ectoderm (or caudal hindbrain) must communicate and work together to achieve complete development of the otocyst. Raj Ladher (Team Leader, Laboratory for Sensory Development) and colleagues at the University of Utah have now determined a role for signals from the third germ layer, endoderm, in the initiation of the inner ear.
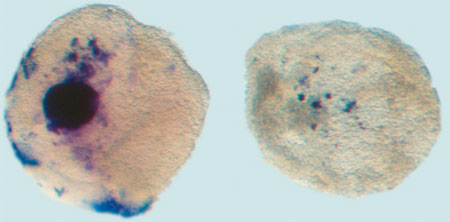 |
Tissue that forms the inner ear expresses the transcription factor Pax2 (left); in tissues where Fgf8 is inhibited in the endoderm, the inner ear as marked by Pax2 expression does not form (right). |
The study of ear development reveals great diversity in molecular agents across taxa, although most of that variety is confined to the FGF family of secreted proteins and receptors. In the chicken embryo, signaling by FGF19 from the cranial mesoderm induces the expression of WNT8c and FGF3 in the neural ectoderm, which then cooperatively induce the otic placode to form in a nearby non-neural ectodermal region. The mouse achieves the same ends by different means, using mesodermal FGF10 and FGF3 in the hindbrain to instruct the placode to form.Zebrafish embryos, meanwhile, take a third route, utilizing FGF3 and FGF8 to engage and regulate otic induction. This last example sparked Ladher’s interest, as there had been no reports of Fgf8 expression in the parotic regions of either chicken or mouse, and, with his colleagues in Utah, he began to investigate the possible involvement of FGF8 in the induction of the ear in these species.
Tissue ablation studies in chick showed that endoderm makes a contribution to the initiation of otic development, which subsequent investigations indicated was due to its induction mesodermal Fgf19. Looking at in the subjacent endoderm at developmental stages corresponding to the start of the Fgf19 expression, Ladher found that Fgf8 is indeed expressed in patterns suggesting a role in otic induction. The team found that exogenous FGF8 was sufficient to induce mesodermal Fgf19, then demonstrated its necessity by inhibiting Fgf8 expression in vivo using RNAi, which resulted in the downregulation of Fgf19 and failure of otic placode development.
In parallel studies in mouse, Fgf8 was found to be expressed in a pattern consistent with a role in the development of the ear, with transcripts detected in relevant sites and at time-points synchronous to otic placode induction. Gene expression patterns indicated a possible overlap in function between Fgf8 and another FGF family member, Fgf3. Embryos entirely lacking Fgf8 die prior to the initiation of ear development, so the team constructed a model in which Fgf8 expression is dramatically reduced and Fgf3 absent to test the effect on otogenesis. They found that, at these drastically low levels, Fgf8 was unable to support ear development in the absence of Fgf3. This effect, however, did not translate to abnormalities in hindbrain development, which contrasts with the case for zebrafish, in which Fgf3 and Fgf8 also function redundantly, suggesting that in mouse the ear and the hindbrain are determined by different genetic routines. Examining the Fgf3/8 double mutants more closely, the team saw that the phenotypes resembled those of Fgf10 knockouts, a similarity which was substantiated at the molecular level by in situ hybridization studies showing clear reductions in Fgf10 expression in the double mutant mesenchyme.
|