|
|
|
|
|
|
|
|
 |
 |
|
 |
Developmental biology is the study of the processes that allow a single cell ? the fertilized egg ? to give rise to a multicellular organism. Animal species follow a broad range of developmental programs and exhibit varying levels of complexity in their physical organization, but many of the processes of development are essentially common to all animals. This display of biological diversity against a background of widely shared traits and rules is what makes developmental biology endlessly fascinating and rewarding to those who study it.
This page will briefly introduce some of the main processes that guide the egg on its way to becoming a mature individual. The mechanisms and phenomena described are common (but universal); the list is neither exhaustive nor specific to any single species. It should also be remembered that these processes show a considerable degree of overlap and cross-function, and should be thought of as occurring in coordinated and cooperative function with each other, not as linear or distinct. If developmental biology teaches anything, it is that nature is boundless in its ability to compose variations on established themes. |
 |
 |
 |
Francois Jacob once said gThe dream of every cell is to become two cells.h This dream is realized by the process of cell division, in which a cell duplicate its contents in order to provide sufficient materials for the organization and operation of both daughter cells as it splits in two. This occurs in a round of events collectively known as the cell cycle, involving successive stages of rest and activity and resulting in the generation of two cells whose nuclei and inner contents are derived from a single parent. Cell division is important to developmental biology as one of the main means by which animals grow ? consider that the human body begins as 1 cell, but through cell division ultimately comes to comprise around 60,000,000,000,000.
|
Most cell divisions result in the generation of two daughter cells that are fundamentally similar in type and function to their parent. However, some cells are able to give rise to cells of types other than their own. The determination of specific cell identities is known as differentiation. The majority of cells in the adult body are highly differentiated, meaning they have very specialized and rigidly defined structures and biological roles. But some types of cells, such as stem cells and progenitor cells, are less differentiated and capable of producing both copies of themselves and daughter cells with different properties. For example, certain blood progenitor cells can give rise to any of the types of blood cell found in the body. The breadth of a cell's ability to generate cells of other types is known as its differentiative potency. This greater the potency, the wider the variety of cell types that cell can produce. Embryonic cells are considered to be pluripotent, meaning that they can generate all of the types of cells found in the body.
These abilities of cells to reproduce by division and to diversify are critical to establishing the multiplicity and complexity of cellular systems in multicellular organisms. |
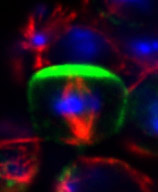 |
|
 |
 |
 |
Cells of different types are able to recognize other cells by using a number of molecular mechanisms that let them discriminate between cells of the same type and others. This is critical for the formation of complex tissues, organs and other bodily structures, as skin cells, for example, need to be able to identify and work together with other skin cells, while at the same time maintaining their functional distinction from muscle, blood and connective tissue cells. |
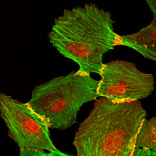 |
Cell recognition is closely linked with cell adhesion. Mechanisms of adhesion exist which effectively function as recognition systems in which cells displaying specific adhesion molecules on their surfaces bind only to other cells that display the same molecules. This sort of mechanism for adhesion allows cells of similar type to form structures composed entirely of only those cells that are necessary to their building and function. One of the most prevalent adhesion mechanisms involves a large family of molecules known as cadherins, each of which binds selectively to cadherins of the same type. By expressing different cadherins on their surfaces, cells are able to establish their own identities and establish and maintain binding relationships with other cells that they encounter. Groups of such cells, linked by adhesion molecules, provide a basis for the formation of higher-order structures, including the neural network, the most complex organismal system known to biology. |
|
 |
 |
 |
Cells are capable of moving in a number of ways, both passive and active. This is important during nearly every stage in development as cells, and structures made up of cells, pass over, under, through or around intervening structures and spaces as they follow their instructions for building an individual body. Developmental biologists are interested in al aspects of cellular locomotion, from the means by which individual cells move, such as by pulling themselves forward by feelers extending from the cell body or crawling over surfaces in a ruffling fashion, to the migration of cells en masse in sheets, clumps and fibrils.
|
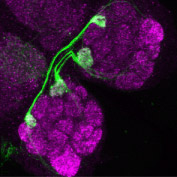 |
|
Cells in the developing body frequently move in a highly directional manner, traversing great distances with a certainty that seems almost conscious. In the nervous system, axons may extend for a meter or more from the body of a neuron to establish a nerve ending at a specific location in the lower leg. Guidance systems help cells find their way by recruiting them to targets using attractant molecules, steering them away from inappropriate destinations, and providing hospitable conditions for their survival once they arrive. In addition to its inherent scientific interest, knowledge of such biological guidance mechanisms may one day provide new means of delivering therapeutic molecules or replacement cells to specific sites in the human body. |
 |
 |
 |
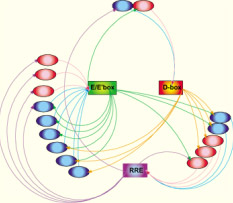
|
Many of the processes described above rely on the ability of cells to communicate with each other via interactions between molecules. Such communications can take place between cells as well as within the environment inside a single cell, or may involve activity on both sides of the cell membrane. The frequency and variety of such molecular signal-based communications is dizzying, with molecules acting individually or in partnerships to trigger, suppress, alter or redirect the activity of other molecules in an as-yet incalculable number of possible combinations. Many signaling events occur as cascades of activity. Molecules originating |
|
within one cell may set off a chain of events that leads to the release of other molecules which may travel across intercellular space to interact with targets even further downstream, perhaps on the surface of another cell, which then trigger or inhibit further activity within the signal-receiving cell. Signaling networks also interact with other networks and respond to their environments, allowing signaling molecules to assume multiple roles in a case-dependent fashion.
One of the most important types of cellular communications is known as signal transduction, in which the signals work to influence the expression of genes. This signaling can be initiated either exterior or interior to the cell, in systems involving signal and receptor molecules whose interactions ultimately work to activate, inhibit or modulate the expression of one or more genes. Signal transduction is a fundamental activity in nearly every aspect of development and is the key that enables the body's cells, which are for the most part genetically identical, to behave in so many different ways and to assume such a vast array of forms and functions. |
 |
 |
 |
The building of an embryo represents the culmination of the results of many smaller scale processes such as those described above, orchestrated to follow a higher-order set of instructions. Whether the embryo is simple, as in the roundworm, or complex, as in the mouse, there are certain features of embryonic development that are shared across the greater part of the animal kingdom. These include the starting point of fertilization, early cell divisions, gastrulation (in which three fundamental cell types or ggerm layersh arise), |
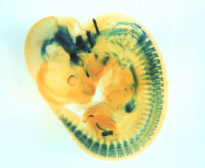 |
and the emergence of the body plan, a basic blueprint that defines the general organizational schemes of an animal's body, including body axes, segmentation, organogenesis and the outline of the nervous system. Embryogenesis is a carefully balanced, multi-level collaboration of molecules, cells and tissues, of signals, codes and processes. Perhaps the greatest fascination, the miracle that compels scientists to study this work of infinite complexity, is that the entire set of instructions needed to perform the work of building a butterfly, shark, person or worm are all contained in the confines of a single cell, the fertilized egg. |
|
 |
 |
 |
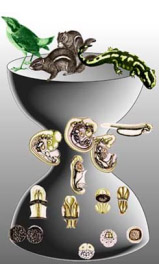 |
Advances in molecular developmental biology and the rapid increase in our knowledge of genomes have allowed us to develop realistic scenarios regarding animal evolution. Surprisingly, it is not only genes that are conserved among animal species, but also the molecular cascades operating in the developmental programs of distantly related animals. These findings have led us to realize the conservative nature of molecular-level patterning systems, which may be related to what is called the developmental constraints that give rise to similarities, or homologies, in structure. The bat wing is merely a modified mammalian arm and could never have evolved without the basic developmental program of the limb.
It is also true, however, that entirely new organs and patterns with no apparent homologous relationship to other structures have often appeared abruptly in the history of animal evolution. Developmental
|
biology also provides an answer to this mystery: since the developmental programs are constructed in hierarchically-organized steps of events, a small shift of function in a few key molecules can exert radical large-scale changes. Genes that encode stress proteins also appear to play an important role in evolution. By buffering mutations in other developmentally important proteins, they have the potential to accrue tremendous numbers of alleles and serve as detonators for new phenotypes.
In these ways developmental biology has begun to provide explanations of the mechanistic aspects of evolution, and molecular-level experiments can now point out where the changes have been introduced for establishment of new phenotypes. |
|
 |
 |
|
|
|
|
|
|
|
|
|